CO2 electroreduction on copper catalysts investigated by in situ X-ray spectroscopy
Juan-Jesús Velasco-Vélez, Qingjun Zhu, Cheng-Hao Chuang, Travis Jones, Dunfeng Gao, Emilia Carbonio, Beatriz Roldan Cuenya, Robert Schlögl, Axel Knop-Gericke
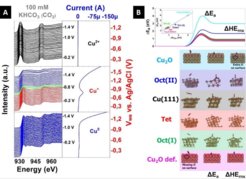
The electrocatalytic route of energy conversionbecomes a key issue because the electricity produced by renewable sources of energy, like solar and wind, can be used to convert CO2 into valuable chemical feedstocks. Over the last decades several materials able to reduce electrochemically CO2 in aqueous solution to produce hydrocarbons have been identified not efficient and stable for practical use. In this direction, copper is unique due to its ability to electro- reduce CO2 to hydrocarbons and alcohols in aqueous electrolytes, as was probed by Hori et al.1 Nevertheless, the selective electroreduction of CO2 into fuels is challenging due to the multiple complex proton-coupled electron transfer steps that must occur.2 This complex network makes the cathodic CO2 reduction reaction (CO2RR) behave with relative low current density and high overpotential as well as electrode deactivation over time. By tracking the electronic structure of the Cu catalysts, using in situ X-ray spectroscopies, we were able to tune and precisely set the initial Cu redox state, such as Cu0, Cu+ and Cu2+, by controlled applied potential protocols.3 Figure 1A shows the variation in the electronic structure that the different catalysts undergo depending on the applied potential revealing the formation of reduced copper during the reactions as well as the formation of a passivation copper carbonate layer onto cupric oxides. The magnitude of this dissociation barrier depends on the degree of surface oxidation and nature of surface defects. Therefore, we calculated the barrier associated with dissociative CO2 adsorption (CO2,ads → COads + Oads) on several copper catalysts, as shown in Figure 1B, indicating that the dissociation barrier is lowered in the presence of missing oxygen on the surface and enhanced in presence of extra oxygen on the surface.
References
1. Y. Hori et al. Chem. Lett. 15(6), 897-898 (1986)
2. R. Kas et al. Chem. Phys. 16(24), 12194-12201 (2014)
3. J.J. Velasco-Velez et al., ACS Sus. Chem. & Eng. 7(1), 1485-1492, (2018)