Research
Bretislav Friedrich's Research revolves around interactions of molecules with and in electric, magnetic, and optical fields. The following specific research topics are currently being pursued:
(1) Supersymmetry and eigensurface topology of molecules in combined electric, magnetic, and optical fields
(2) Stark spectroscopy of molecular complexes created and probed in He nanodroplets
(3) Quantum computing with molecules
(4) Manipulation of molecules by means of external fields
(5) Molecular collisions in fields
(6) Spectroscopy and imaging of molecules in fields
(7) Cold/slow molecules
The research program is partly funded by the DFG.
(1) Supersymmetry and eigensurface topology of molecules in combined electric, magnetic, and optical fields
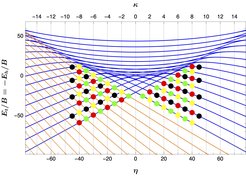
We made use of supersymmetric quantum mechanics (SUSY QM) to find sets of conditions under which the problem of a polar paramagnetic molecule subject to combined electric, magnetic, and optical fields is analytically solvable. The analytic forms of the eigenfuntions made it possible to find analytic expressions for the observables of interest, such as the expectation values of the angular momentum squared and of the orientation and alignment cosines as well as of the eigenenergy. Furthermore, we found that the topology of the intersections of the molecule's eigenenergy surfaces can be characterized by a single integer index whose values correspond to the sets of conditions under which the analytic solutions to the quantum pendulum problem exist. We make use of the Quantum Hamilton-Jacobi (QHJ) theory to investigate conditional quasi-solvability. We derive the conditions of quasi-solvability of the time-independent Schrödinger equation as well as the corresponding finite sets of exact analytic solutions. We do so for of a quantum symmetric top subject to combined electric fields (symmetric top pendulum) as well as for its anti-isospectral hyperbolic counterpart. An examination of the algebraic and numerical spectra of these two systems reveals mutually closely related patterns. The QHJ approach allows to retrieve the closed-form solutions for the spherical and planar pendula and the Razavy system that had been obtained in our earlier work via Supersymmetric Quantum Mechanics as well as to find a cornucopia of additional exact analytic solutions.
Collaborators:
Burkhard Schmidt (Berlin), Simon Becker (Cambridge), Konrad Schatz (Berlin).
(2) Stark spectroscopy of molecular complexes created and probed in He nanodroplets
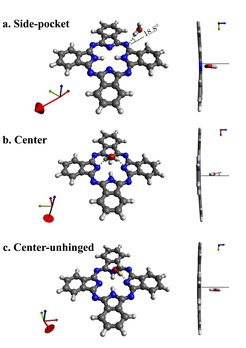
The objective of the work pursued herein is to examine the binding by dispersion forces of van der Waals complexes. The experimental technique of choice is high-resolution electronic spectroscopy capable of resolving the structure of the van der Waals complexes of well defined stoichiometry generated in superfluid helium droplets at a temperature of only 0.38 K. The key innovation with respect to our previous work is the implementation of Stark spectroscopy. Stark spectroscopy holds the promise of resolving various isomeric variants of the complexes that form in superfluid helium nanodroplets. Thus the project contributes to structural studies in general and the quantification of dispersion interactions in particular by investigating a series of prototypical van der Waals clusters. By making use of optical spectroscopy, the investigations also provides access to electronically excited states of van der Waals clusters. The project has unfolded from the implementation of lock-in enhanced Stark spectroscopy as an experimental tool for unravelling the configurations of van der Waals clusters and thereby of the underlying dispersion forces. A simultaneous theoretical analysis is beingused to affirm the structural information on the clusters as revealed by the optical Stark spectra. Our experimental and theoretical approach will be easily applicable to any other types of van der Waals complexes amenable to optical spectroscopy.
Collaborators:
Alkwin Slenczka (Regensburg), Mallikarjun Karra (Berlin).
(3) Quantum computing with molecules
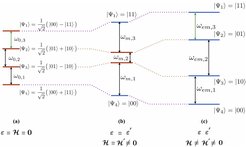
Since the original proposal by David DeMille, arrays of ultracold polar molecules have been counted among the most promising platforms for the implementation of a quantum computer. The qubit of such an array is realized by a single dipolar molecule entangled via its dipole-dipole interaction with the rest of the array's molecules. The electric dipole interaction ensures that each qubit is interacting not only with its neighbors but also with every other qubit in the system. Previous studies of entanglement of electric dipoles have not adequately considered how the external electric field affects both the qubit states and the dipole-dipole interaction. In our current work we consider entanglement for ultracold polar and paramagnetic molecules trapped in a two-dimensional optical lattice in congruent electric and magnetic fields. The presence of the unpaired electron in a, say, doublet Sigma molecule can be used for encoding quantum information as in other spin-1/2 particles. Understanding the dynamics of spin excitation transfer between molecules in the combined fields will allow us to tune the entanglement in such a system. We are also investigating methods of designing quantum logical gates, one-qubit gates, and two-bit quantum gates (such as the CNOT gate) for molecular dipole arrays.
Collaborators:
Ketan Sharma (Berlin), Mallikarjun Karra (Berlin), Sabre Kais (West Lafayette), Dudley Herschbach (Cambridge, MA).
(4) Manipulation of molecules
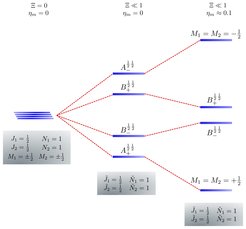
Directional states of molecules are at the core of all methods to manipulate molecular trajectories. This is because only in directional states are the body-fixed multipole moments 'available' in the laboratory frame where they can be acted upon by space-fixed fields. In the case of polar molecules, the body-fixed permanent dipole moment is put to such a full use in the laboratory by creating oriented states characterized by as complete a projection of the body-fixed dipole moment on the space-fixed axis as the uncertainty principle allows. In the past, in order to achieve a high degree of orientation, one had to rely on special properties of particular molecules. The need for such a reliance has been superseded by the development of a versatile techniques that combines a static electric field with a nonresonant optical field. The combined fields give rise to an amplification effect which occurs for any polar molecule, as only an anisotropic polarizability, along with a permanent dipole moment, is required. This is always available in polar molecules. Thus, for a number of molecules in their rotational ground state, a very weak static electric field can convert second-order alignment by a laser into a strong first-order orientation that projects about 90% of the body-fixed dipole moment on the static field direction. If the polar molecule is also paramagnetic, combined static electric and magnetic fields yield similar amplification effects, which are indeed the subject of our present work.
Collaborators:
Ketan Sharma (Berlin.
(5) Collisions of molecules in electric, magnetic, or radiative fields
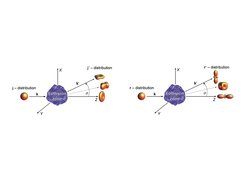
Collisions of molecules in electric, magnetic, and radiative fields are nearly ubiquitous in nature as well as in the laboratory. Molecules colliding in the Earth's atmosphere or in interstellar space are commonly subject to magnetic and radiative fields; in the laboratory, collisions in fields appear with particular prominence in stereodynamics, coherent control, and molecular trapping and cooling. Molecular collisions in fields have been the subject of a number of theoretical studies. However, analytic models of such collisions are scarce and limited to the collision regime near the Wigner limit. Recently, we have developed an analytic model of state-to-state rotationally inelastic collisions of atoms with molecules in fields, applicable at thermal and hyperthermal collision energies. The model, based on Fraunhofer scattering of matter waves, is inherently quantum and makes it possible to separate dynamical and geometric effects in collisions. Our current effort is being directed at identifying form factors in vector correlations observed in state-of-the-art experiments and/or full-fledged computations.
Collaborators:
Mikhail Lemeshko (IST Austria), Marcelo de Miranda (Leeds), Javier Aoiz (Madrid), Millard Alexander (Maryland).
(6) Spectroscopy and imaging of molecules in electric, magnetic, or radiative fields and their combinations
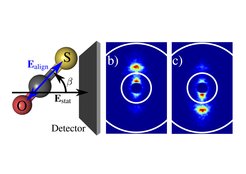
Oriented states have a unique spectroscopic behavior, due to their sui generis energy level patterns and modified Hönl-London factors. Our effort is directed towards identifying oriented states of molecules in the combined fields, characterizing their spectroscopic response in both the time and frequency domain, and devising means to enhance the spatial resolution of molecular imaging.
Collaborators:
Alkwin Slenczka (Regensburg), Burkhard Schmidt (Berlin), Henrik Stapelfeldt (Aarhus), Rosario Gonzalez-Ferez (Granada).
(7) Cold molecules
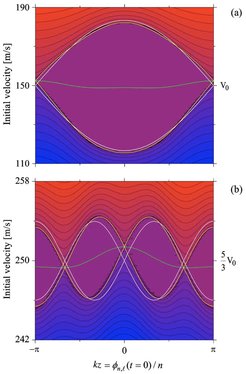
Over the last two decades, atomic and molecular physics has come into a spectacular bloom, much of which sprang from the newfashioned techniques to translationally cool (or slow down) gaseous atoms and molecules. The study of slow atoms and molecules, which ensued, has led to uncharted territories - not just of atomic and molecular physics, but of physics and chemistry at large. We've been engaged in developing techniques of slowing and trapping molecules based on time-varying inhomogeneous electric fields, either due to a pulsed laser or due to switched electrostatic fields. So far we worked out an analytic model of the Stark deceleration process and proposed an AC trap for high-field seeking molecules, which has meanwhile been implemented.
Collaborators:
Gerard Meijer (Berlin), John Doyle (Cambridge, MA), David Patterson (University of California at Santa Barbara).