How Local Solid State Chemistry Drives and Limits the Performance of Lanthanum Strontium Manganite-Based Solid Oxide Electrolysis Cells
Solid Oxide Electrolysis Cells (SOECs) are efficient electrochemical devices that convert electrical energy into chemical energy by splitting water into hydrogen and oxygen, or carbon dioxide into carbon monoxide and oxygen. A team of scientists from multiple institutions, led by researchers from the Fritz Haber Institute and the FZ Jülich, has provided unprecedented insights into the atomic-scale changes affecting SOEC performance. The team studied the aging and structural transformations of the electrode material Lanthanum Strontium Manganite (LSM) and the electrolyte Yttria-Stabilized Zirconia (YSZ) during operation. Their findings highlight how local solid-state chemistry, such as cation diffusion and secondary phase formation, influences both the performance and stability of SOECs, paving the way for strategies to improve the durability and efficiency of these cells.
Key features of Solid Oxide Electrolysis Cells and their operating mechanism
Solid Oxide Electrolysis Cells (SOECs) are a type of electrochemical cell used to convert electrical energy into chemical energy by splitting water (H2O) into hydrogen (H2) or carbon dioxide (CO2) into carbon monoxide (CO). They operate at high temperatures (typically 600–900 °C) and use a solid oxide ceramic material as the electrolyte, which is why they are called "solid oxide" electrolysis cells. Due to their operation at high temperatures, SOECs can achieve high efficiencies in producing hydrogen or other gases and can be integrated as intermittent energy storage from renewable energy sources such as solar or wind. However, their performance under such high temperatures is impacted by the chemical interactions and associated structural changes that take place during operation. Thus, their lifespan is constrained by rapid deactivation and the atomic-scale transformations responsible for this degradation remain poorly understood.
Atomic-Scale Changes Affecting SOEC Performance and Stability
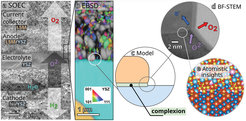
A groundbreaking study, led by researchers from the Theory and Inorganic Chemistry departments at the Fritz Haber Institute, in collaboration with scientists from the Forschungszentrum Jülich GmbH, has shed new light on the impact of local solid-state chemistry on the efficiency of SOECs. By combining high-resolution scanning transmission electron microscopy with first-principles and force-field-based atomistic simulations, they were able to investigate reaction-induced changes at the atomic scale in a SOEC operated for 550 hours. In particular, they focused on how the structure of the Lanthanum Strontium Manganite (LSM) and Yttria-Stabilized Zirconia (YSZ) regions, as well as their interface, change over time.
The LSM and YSZ regions are essential for SOECs, with LSM acting as an efficient electrode material for electron conduction and YSZ offering high ionic conductivity to facilitate oxygen ion transport. Combined, these materials enable the formation of oxygen gas in the SOECs during electrolysis.
One key finding is that cations diffuse significantly between the different material regions, leading to the formation and growth of new localized structures. These include small domains of solid solutions, secondary phases, or defects in the YSZ and areas with mixed ion and electron conduction at the interface and in the LSM. Depending on their location, these changes can either enhance or impair performance: on the one hand, improving electron and ion conductivity is crucial for the functionality of the cell, while on the other hand, oxygen ion transport becomes restricted. This interplay underscores the significance of atomic-scale structuring in the performance of SOECs.
Towards Advancing SOEC Performance: Integrating Atomic-Scale Insights to Enhance Durability and Efficiency
Overall, this approach underscores the value of integrating atomic-scale experimentation with theory and encourages the development of aging and operando experiments to track structural changes in interfacial regions, demonstrating their importance for understanding performance. Ultimately, this research enhances our understanding of the degradation mechanisms in SOECs and paves the way for the development of nanoscopic, rational interface designs to create more efficient and durable cells.
“Diffusional solid-state processes are slow. The study impressively shows that long-term measurements are essential in order to study and understand the deactivation behavior of SOECs.", said Dr. Thomas Lunkenbein, corresponding author and group leader for Electron Microscopy in the Department of Inorganic Chemistry at the Fritz Haber Institute.
Dr. Hanna Türk, first author and member of the Theory Department at the Fritz Haber Institute, who is currently pursuing a Postdoc at EPFL, points out how the study suggests a promising future for the SOECs themselves: "This is the first time we have been able to examine the properties and aging processes of the solid-solid interface in such detail. This breakthrough allows us to identify potential causes of deactivation and now enables us to strategically explore methods to extend the cells’ lifespan.”