
Light Matter Interactions in Energy Materials
Kick Group
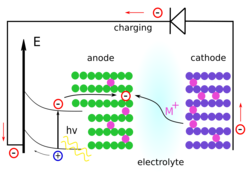
We employ state-of-the-art electronic structure methods and develop new approaches where existing methods fall short to describe light-matter interactions with a particular focus on energy materials. By using both established and novel methods, we study prototypical compounds to build an atomistic understanding of the charge storage mechanisms in these materials.
Such systems are inherently complex. Excitonic states, quantum confinement, electron localization, and charge carrier dynamics are crucial to the performance of these novel materials. Additionally, charge storage must occur with high efficiency to suppress competing processes, such as non-radiative decay, to prevent device degradation. A detailed understanding of such interactions would allow us to design novel energy materials with superior properties. In particular, we are interested in materials which can combine both light-harvesting and energy storage properties in one single device. This would make solar cells obsolete and would directly result in very simple systems which could be a key component in the pursuit of micro to nano devices. For example, envision nano batteries which can be charged directly by sunlight, without external solar cells, or artificial mitochondria which harness and store solar energy to perform complex catalytic reactions autonomously day and night. (https://www.fkf.mpg.de/8175585/2024_02_Gouder)
The second focus of our group lies in the development of efficient methods for the description of excited state phenomena in large and largest systems. We are developing computational tools and methods that provide a hierarchical set of approximations to efficiently address the challenge of calculating a large number of excited states when standard methods become computationally prohibitive. We tackle the issue that the number of relevant states increases rapidly with system size. For molecules adsorbed on surfaces, quantum dots, nanoplatelets, and other nanoscale light-harvesting devices. Here, surface excitons increase super-linearly, and bulk, surface, and molecular states mix, forming a quasi-continuum within a small energy range, necessitating the evaluation of many states. Moreover, the lowest excitations might not be relevant, as they may stem from surface excitations rather than the desired excitonic transitions. Often, hundreds or even thousands of transitions must be calculated before one finds the relevant ones. Our computational methods contribute to efficiently addressing the enormous amount of relevant states. (https://www.nature.com/articles/s41467-024-52368-5)
Methods:
Density Functional Theory
Time-Dependent Density Functional Theory
Self-Interaction Correction Methods
Model Hamiltonians
Machine-Learning Techniques