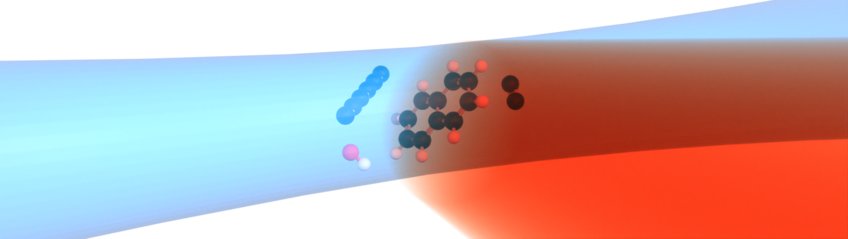
Research
The interstellar medium—where cold, isolated gas phase molecules are the norm rather than the exception—provides very different conditions from those in the condensed phase. These conditions allow for the occurrence of special fluorescence processes that can help in stabilizing molecules after chemical reactions and may explain so far unassigned emission lines observed by space telescopes and ground-based observatories, such as the “unidentified infrared emission” or the “extended red emission”. Two important fluorescence processes that can occur in larger isolated molecules are recurrent fluorescence, a special form of electronic fluorescence coupled with inverse internal conversion, and infrared fluorescence from highly excited vibrational states.
Recurrent Fluorescence
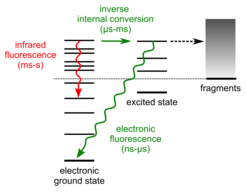
Already in 1988, recurrent fluorescence (or Poincare fluorescence) has been proposed to play a role in the stabilization of highly isolated polyatomic molecules. It involves inverse internal conversion (e.g. from a vibrationally excited state of the electronic ground state to an electronically excited state) immediately followed by electronic fluorescence. Typically, internal conversion is considered to be an irreversible process in the direction of the lower-lying electronic state, reflecting the significantly larger density of states at a given energy. However, statistics also allow for the reverse process, leading to a small rate of energy transfer back to the excited state, which can become relevant for isolated molecules on sufficiently long timescales. For molecules formed in interstellar space, this fluorescence mechanism may therefore present an effective radiative cooling channel.
Only recently, experiments performed on ions held in electrostatic ion beam traps and storage rings have indeed shown the emission of visible light from hot C4–, C6– and naphthalene+ molecules on timescales from 10 µs to 1 ms. These effective emission timescales are much longer than regular fluorescence lifetimes of those molecules and are thus believed to originate from recurrent fluorescence. Similar radiative cooling timescales have been inferred for other carbonaceous molecules, including polycyclic aromatic hydrocarbons and fullerenes, based on more indirect techniques—suggesting the presence of recurrent fluorescence in many other interstellar molecules.
“Detection of Recurrent Fluorescence Photons”, Y. Ebara et al., Phys. Rev. Lett. 117, 133004 (2016).
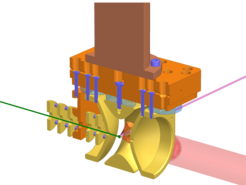
Our goal is to set up an experiment for studying the emission resulting from recurrent fluorescence with vibrational state resolution on the relevant timescales up to several milliseconds. This includes the measurement of dispersed emission spectra and radiative lifetimes of trapped molecular ions prepared in well-defined initial quantum states (in particular vibrational states). This is achieved using cryogenic cooling and UV/Vis laser excitation. We are therefore designing a customized cryogenic ion trap with optical access that will allow us to store a sufficient number of cold ions to study the recurrent fluorescence process in great detail. The experiment will further have access to a beamline of the FHI infrared free-electron laser, which will give us the opportunity to additionally use IR-UV/Vis double-resonance excitation for preparing the initial state of the molecule.
Infrared fluorescence from highly vibrationally excited states
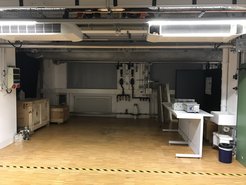
The fast internal conversion in systems that show recurrent fluorescence automatically produces highly vibrationally excited molecules. Infrared fluorescence from these highly excited vibrational states therefore presents another important radiative cooling channel that may compete with recurrent fluorescence in infrared-active molecules. A long-term goal of our research is therefore to extend our state- and time-resolved fluorescence measurements towards mid-infrared wavelengths. As traditional infrared detectors typically lack the requirements for efficient single-photon detection in this spectral region, we plan to utilize superconducting detectors. Sensitivity at wavelengths up to 10 µm has already been demonstrated for superconducting nanowire single-photon detectors (SNSPDs) and active development towards even more demanding mid-infrared applications is ongoing at the NIST in Boulder, Colorado.
SNSPDs have already been successfully used in spectroscopy for mid-infrared emission experiments of CO molecules attached to a NaCl crystal surface. There, fascinating vibrational energy transfer phenomena between the CO molecules and from CO to the weakly interacting NaCl substrate have been observed. Here, we would like to make use of the exceptional properties of these detectors, in particular their low dark count rates and high sensitivity, to probe the internal energy transfer dynamics of isolated gas phase molecules.
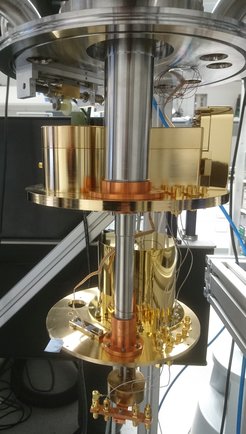