Cobalt in the OER and Isopropanol Oxidation
T. Götsch, A. Klyushin, T. Lunkenbein, R. Schlögl, A. Knop-Gericke
While iridium oxide lies at the tip of the volcano plot for the oxygen evolution reaction,1 its use is limited by reasons of economics and availability, as well as its instability in alkaline conditions.2 Thus, it is desirable to replace these expensive and rare precious metals that cu electrocatalysts by more abundant first row transition metals such as cobalt.3 We have recently begun investigating Co- based materials for use in alkaline water splitting using near-ambient pressure XPS. However, the change from acidic to alkaline conditions poses new challenges such as material incompatibilities and the suitability of reference electrodes for the new chemical environment. Moreover, cobalt is prone to dissolve at non-basic pH values,4 rendering the sample preparation process more difficult. First preliminary results of a graphene-covered sputtered Co film that could be kept stable by never exposing it to pH values below 13, measured in our Nafion cell with a confined electrolyte (100 mM KOH), are given in Figure 1A and B, where a continuous oxidation can be observed in the cobalt L3 edge (A), while the position of the main peak in the O-K edge (B) shifts with the potential.
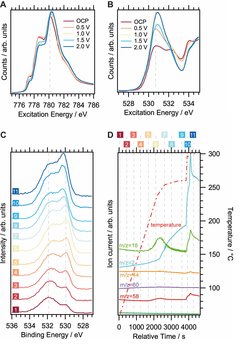
In addition to being in the process of constructing a quasi in situ cell for TEM investigations of these systems, we will further expand these studies to encompass different cobalt-containing oxides such as different spinels or perovskites. As the latter are ionic conductors, they are also suitable for investigating high temperature water splitting in solid oxide electrolysis cells. For this purpose, we are currently developing an operando solid oxide fuel/electrolysis cell setup with separated anode and cathode compartments that will be compatible with our NAP-XPS systems and our ESEM.
The application of these systems is not limited to electrochemistry, but oxidic cobalt compounds can be utilized in alcohol oxidation reactions as well. In a first step to eventually approaching liquid phase heterogeneous catalysis in our synchrotron-based XPS systems, we have performed gas phase isopropanol oxidation and decomposition reactions to acetone over cobalt(II,III) oxide nanoparticles. These operando measurements were completed at the new UE48 beamline at BESSY II and O 1s spectra acquired during isopropanol decomposition are given in Figure 1C. Figure 1D shows the accompanying product evolution as detected using mass spectrometry and the colored numbers label the points in time when the respective spectra were taken. At first, the higher-binding-energy component, which can be related to isopropanol species, shift with temperature, whereas the lattice oxygen (below 530 eV) shifts at the highest temperatures.
(1) Seh, Z. W.; Kibsgaard, J.; Dickens, C. F.; Chorkendorff, I.; Nørskov, J. K.; Jaramillo, T. F. Combining Theory and Experiment in Electrocatalysis: Insights into Materials Design. Science 2017, 355 (6321), eaad4998. https://doi.org/10.1126/science.aad4998.
(2) McCrory, C. C. L.; Jung, S.; Peters, J. C.; Jaramillo, T. F. Benchmarking Heterogeneous Electrocatalysts for the Oxygen Evolution Reaction. J. Am. Chem. Soc. 2013, 135 (45), 16977– 16987. https://doi.org/10.1021/ja407115p.
(3) Lu, F.; Zhou, M.; Zhou, Y.; Zeng, X. First-Row Transition Metal Based Catalysts for the Oxygen Evolution Reaction under Alkaline Conditions: Basic Principles and Recent Advances. Small 2017, 13 (45), 1701931. https://doi.org/10.1002/smll.201701931.
(4) Garcia, E. M.; Santos, J. S.; Pereira, E. C.; Freitas, M. B. J. G. Electrodeposition of Cobalt from Spent Li-Ion Battery Cathodes by the Electrochemistry Quartz Crystal Microbalance Technique. J. Power Sources 2008, 185 (1), 549–553. https://doi.org/10.1016/j.jpowsour.2008.07.011.