Find the gap - Behind the scenes of basic research
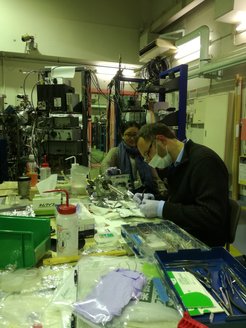
It's 11 pm. My eyes red and tired, I look at a display that spits out new data points every 20 seconds. A long day lies behind me and my colleagues, full of repairs, sample preparations and short trips to the campus kiosk, where we stock up on coffee for our long shifts. We are on a research visit at the University of Tokyo’s ion accelerator laboratory. Of course, we do not work in the accelerator itself. We are only allowed in the control room. The radiation produced by our experiment just beyond the pink lead walls would be absolutely hazardous to our health. Our search for the smallest of all atoms – hydrogen – has led us here. The most common element in our universe is so tiny that it is difficult to detect by conventional methods. In Tokyo, we are using a special form of nuclear fusion to find out whether hydrogen can be stored in the material cerium oxide.
Why? We don't know yet. Unlike in applied research, which ends with the development of a new product or process, we are at the very beginning. We are undertaking basic research. Because even if a glance at conventional textbooks suggests the opposite, at the level of atoms we are far from knowing everything about the world around us. We want to find out whether hydrogen can be stored in the solid cerium oxide, because this knowledge could lay the foundation for future applications, wherever hydrogen is used, from the production of medicines to new power engines.
A molecular sponge cleans air – can it also store hydrogen?
What we know: Cerium oxide can store oxygen. Built from two parts oxygen and one part cerium, the solid is a material with amazing capabilities. When cerium oxide is heated to high temperatures, it readily releases oxygen atoms from its atomic lattice to the environment without changing its shape. What remains are empty spaces, smallest gaps in the material, like in a sponge. For its generosity in handling oxygen, ceroxide is popular as an auxiliary material in many reactions. For example, it can be found in almost every car, where it ensures that there is enough oxygen to convert toxic exhaust gases into non-toxic exhaust gases in the car catalyst.
While this superpower of ceroxide is well researched, we know little about its interaction with other substances. Would it be possible that it could store hydrogen like a sponge, too? If so, it would open new doors: In a reaction where hydrogen is needed, cerium oxide could first absorb hydrogen and then release it when needed. This would eliminate intermediate steps such as the supply and removal of hydrogen gas, and the corresponding industrial processes could be more energy- and resource-efficient.
There is already a significant indication of a special interaction. A research team at ETH Zurich reported in 2012 that cerium oxide facilitates the addition of hydrogen atoms to small organic molecules by an astonishing degree. What is it about the special relationship between hydrogen and cerium oxide?
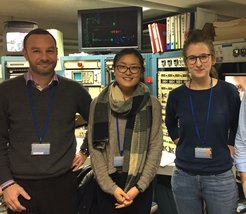
An unexpected Mona Lisa
To answer this question, we conduct an analysis that uses nuclear fusion. For this we need special technical equipment, which, apart from the laboratory in Tokyo, only a few other research institutions worldwide provide. Above all, we need an experimental setup that can accelerate ions to extremely high energies. At the speed the ions reach, they would travel from Tokyo to Berlin (where I work at the Fritz Haber Institute of the Max Planck Society), in under three seconds.
If one of these fast ions now meets a hydrogen atom, both merge to form a new atomic nucleus. This atomic nucleus immediately breaks down into smaller components, releasing gamma rays that have so much energy that they would easily penetrate granite. Only lead walls can stop the high-energy radiation. Behind such walls, in the control room of the laboratory, the amount of radiation tells us how much hydrogen is present in a sample. The method is so exceptionally accurate that Markus Wilde, Associate Professor of Physical Chemistry at the University of Tokyo, affectionately calls it the "Mona Lisa of analytical methods" - a phrase coined by French pysicist Georges Amsel in the 1970s.
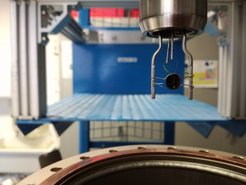
The sample is no less extraordinary than the experiment. It is, in fact, almost perfect. While natural solids consist of a chaotic landscape of corners, edges, craters and hills, our ceroxide surface is flat and ordered. Every atom is in its original place so that we can draw unaltered conclusions about the position of possible hydrogen deposits. To keep this perfect surface clean, it is in a vacuum. This requires an enormous technical effort. Our sample, just about half the size of a copper coin, is located in a two-meter-high steel colossus from which numerous pumps remove all gases around the clock. The effort pays off: Around our sample there is nothing but a few molecules. Just as if it were floating next to the ISS in empty space.
Storing hydrogen - in the smallest Emmental cheese in the world
In order to learn about the storage properties of cerium oxide, we add hydrogen molecules to it, each of which is made up of two hydrogen atoms and is usually very reluctant to form chemical bonds. The reason for this is the strong bond between the two atoms. In their ground state they are so closely linked that they have no interest in reacting with other substances. In order to store hydrogen atoms, cerium oxide must be able to build up an even stronger bond with the individual atoms and thus split the molecule. If the cerium oxide sample does not manage to do this, storage is not possible. This is exactly what we painfully experience in the first weeks of our experiments. Only in exceptional cases does the cerium oxide surface succeed in binding hydrogen. The oxide is simply not reactive enough.
What to do? The solution is to activate the inert ceroxide, to make room! By heating it up, we release oxygen from the atomic lattice and thus create tiny gaps in the material. After heat treatment, our sample looks – on a microscopic level – like a prize-winning Emmental cheese. It also has another special feature, the fundamental importance of which will be revealed later: the oxygen atoms have left reactive electrons around the holes.
After several attempts, on the last day of our research stay, we finally carry out the decisive experiment. Indeed, when we treat the cerium oxide sample with hydrogen, the results are astonishing. Our analysis detects hydrogen not only on the surface but also in the depths of the cerium oxide. So there it is, cerium oxide can store hydrogen! We breathe a sigh of relief. The weeks of experimentation have paid off.
What is happening inside? Computer experiments on the digital twin
![And this is how the hydride in the cerium oxide could look like (left picture): A gap has formed in the lattice of oxygen (red) and cerium (blue) ions in which hydrogen can be stored as a hydride. When the system is heated, the hydrogen is released again and migrates to the surface where it is available for reactions (right picture). Source: arXiv.1904.13200 [cond-mat.mtrl-sci]](/476474/original-1599579265.jpg?t=eyJ3aWR0aCI6MjQ2LCJvYmpfaWQiOjQ3NjQ3NH0%3D--494d16c3ceb3b9a0e17991c779266d6f34d51ae0)
An explanation of what happens inside the ceroxide is provided by our colleagues from the team around Prof. Joachim Sauer at the Humboldt University in Berlin. In elaborate simulations, the quantum chemists reconstruct our cerium oxide sample on the computer – with and without oxygen holes – and calculate the stability of the hydrogen in this digital copy. Such cooperation between experiment and theory is now standard practice when new reaction mechanisms are to be formulated in chemistry. It will soon become clear that the storage of hydrogen in cerium oxide is only possible thanks to the reactive electrons that keep the oxygen holes on standby.

Electrons are the actual driving force in chemistry. They pass from one atom to the next when common salt is formed from the elements sodium and chlorine. Electrons ensure that atoms bind together and become molecules, like an atomic glue that connects the smallest building blocks of life. Electrons are behind the photosynthesis of the green plants on the windowsill, the explosion of firework rockets – and behind the storage of hydrogen in cerium oxide. My other experiments, back in Berlin, confirm their important role: hydrogen steals the electrons that the oxygen has left behind in the gaps in the cerium oxide. The electron-rich form of hydrogen that is created in this process – a hydride – is particularly reactive and rarely found elsewhere. In fact, this form of hydrogen is so rare that we can hardly use it in industrial processes. Our discovery of the storage properties of cerium oxide could change this.
What is the next step? From basic to applied research
This hydride could well be used in the future. It is conceivable, for example, that the inexpensive and light ceroxide could be used as an intermediate storage medium for hydrogen in fuel cells. Right now, this is all just speculation. Which developments will be made possible by the newly found superpower of ceroxide will reveal itself only when applied research takes over the baton.